Nearshore
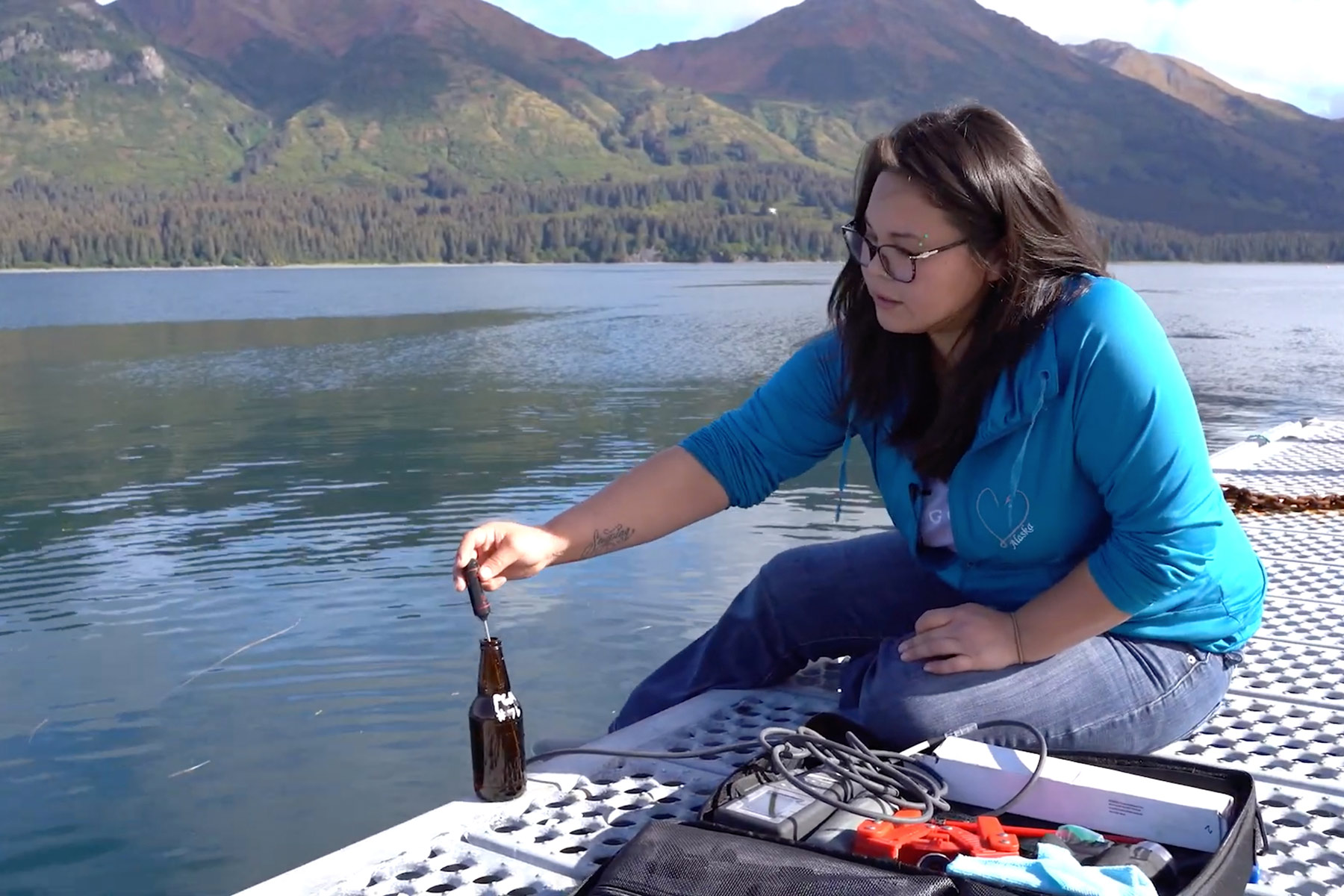
Naomi McMullen of Port Graham takes a water sample on the community dock. Photo from CRRC.
What We Know
Research on nearshore ocean acidification conditions in Alaska is relatively new, but has expanded significantly in the past few years thanks to Tribal community sampling, shorebased continuous monitoring, and an instrumented state ferry. Current research efforts are focused on documenting baseline conditions by recording how corrosive the waters are right now and learning how their pH levels fluctuate on a seasonal basis. With time, we will be able to identify trends, the role of local influences, and eventually the rate of change due to ocean acidification. Detecting the rate of change takes much longer in the nearshore than in the open ocean because of higher natural variability.
Why are nearshore conditions so variable?
Nearshore environments are subject to physical (temperature and salinity) and biological (photosynthesis/respiration) factors that influence the magnitude of chemical changes across daily, weekly and seasonal time scales. In Alaska, unique seasonality, including extended periods of sunlight, proximity to glacial input, and tidal forces can further modify chemical dynamics in the nearshore. Collectively, these factors can lead to significant chemical fluctuations in seawater.
What are we seeing so far?
So far, we know that the nearshore is experiencing corrosive water in some parts of the state during certain times of year. The data indicate a general seasonal trend where the water is more corrosive (lower ΩArag) in fall and winter due to strong storms that mix the water column, and less corrosive (higher ΩArag) during summer months due to the role of photosynthesizing plankton (which take up CO2 and release oxygen during photosynthesis) and glacial and snow melt.
Community Sampling
Over 20 Tribal communities across Alaska are taking weekly water samples to develop baseline data on ocean acidification in a citizen science effort that is unique in the U.S. The water samples are used to build an understanding of current local conditions in the nearshore environment, seasonal changes, and potential natural influences. By creating a consistent time series, communities can better understand the current water chemistry in areas that are important for harvesting shellfish and other species they rely on for their subsistence way of life. Learn more about the Community Sampling Program here.
Photo Gallery
↓Click to enlarge↓
Conditions in locations around the state are starting to come into view with an increase in data collection and analysis. For example, conditions in Kachemak Bay suggest it could be a refuge from ocean acidification while the waters around Juneau appear to be an ocean acidification hotspot. As current monitoring efforts build time series of nearshore data and the community sampling program expands, more gaps will be filled along the coast of Alaska.
Southcentral Alaska (Resurrection Bay, Prince William Sound)
Initial findings show:
- In winter, the data from the Seward Burke-o-Lator tracked similarly to the GAKOA mooring at the mouth of Resurrection Bay. This is likely due to increased storms this time of year and better mixing in the water column. Alutiiq Pride responds to this increase in corrosivity (ΩArag ~1.2) detected by the Burke-o-Lator by adding soda ash to hatchery waters from November through April in order to buffer the corrosivity for developing shellfish.
- In summer, the Seward Burke-o-Lator and GAKOA mooring data tracked differently. Researchers expect this is due to high rates of primary production (growth of phytoplankton) at the surface which results in a draw-down of CO2 into the water column. It’s also believed that warmer temperatures penetrating deeper into the water column in the summer are causing the variability in CO2 at Alutiiq Pride as warmer water holds less gas.
- Community sampling data collected in Seldovia were consistent with the winter data from both Seward and GAKOA, and summer trends at GAKOA.
The comparison between the Burke-o-Lator data and the GAKOA mooring show the Burke-o-Lator can reveal trends applicable beyond the Alutiiq Pride hatchery and may be able to track the conditions of the upper water column elsewhere in the region during winter – the time period when perhaps the ocean acidification trend may be easier to identify from the natural background variability.
Kachemak Bay
It is important to note that Kachemak Bay is home to a number of successful oyster farms whose production may be impacted by future ocean acidification. As sensors continue to collect data, researchers will be able to expand on this time-series to better determine and quantify anthropogenic (human-caused) CO2 intrusion in Kachemak Bay in the coming years.
Kodiak Nearshore
The data collected as part of this program are considered baseline data because they allow researchers to measure environmental conditions that have never been monitored to this extent on Kodiak Island. Tribal communities can then use these data to better understand, adjust, and adapt to changing water quality conditions that can impact subsistence resources, economic development, and overall livelihoods. Researchers at the Kodiak Laboratory also use these data in their multi-year experiments designed to assess the physiological response of early life history stages of commercial crab species, while estimating the potential for acclimation and adaptation to the marine chemistry changes expected with ocean acidification.
Southeast Nearshore
Here are a few of the takeaways from the Columbia study. For more information about current and future nearshore conditions, visit the Southeast Alaska Regional Conditions page.
- The Alexander Archipelago exhibited large variability in acidity and corrosivity relative to other areas along the Inside Passage. This was especially true for northern Southeast Alaska (Chatham Strait, Frederick Sound, Stevens Passage, Peril Strait, and Lynn Canal).
- Contrasting with these highly variable areas, Sergius Narrows and Wrangell Pass had low variability owing to the influence of persistent tidal mixing.
- In terms of severity, Lynn Canal and Wrangell Narrows were quite corrosive (severe Ωarag values) but less acidic (less severe pH).
- Areas exposed to the open continental shelf, such as Dixon Entrance and near Sitka, generally had less severe and variable acidity and corrosivity (pH and Ωarag) compared to confined waters along the Inside Passage.
Arctic Lagoon
Several illuminating trends have emerged in the data.
- Kaktovik Lagoon appears to exhibit some of the most dramatic fluctuations in pH (acidity) ever recorded. Gaining a better understanding of these dynamic conditions will lend insight into the community structure of the resident species in the lagoon, which includes a rich diversity of anadromous fish. Researchers are interested in studying the mechanisms of adaptation that local species have used to survive these dramatic hourly fluctuations.
- The relationship between seawater pH (acidity) and salinity (salt content) ranges across a spectrum that changes by season. When the seawater is ice-free, the relationship between these two variables is either positive or neutral, while the ice-covered season resulted in a negative correlation. This isn’t what researchers were expecting to find. This deviation from the norm suggests that the physical and biological processes that influence seawater acidity (pH) are dynamic and susceptible to future change – particularly from freshwater runoff and shifts in the way organisms who live there feed and produce CO2.
- Bodies of water or other biomes on earth can act either as CO2 “sinks” by absorbing CO2 from the atmosphere, or as “sources” by emitting CO2 into the atmosphere. The world’s oceans have generally acted as carbon sinks, where the absorption of additional carbon causes an increase in acidity and results in the process we know as ocean acidification. However, depending on local or regional chemical and biological processes, bodies of water can also be sources of CO2 through outgassing – particularly nearshore estuaries . The Western Arctic has long been considered a large sink for atmospheric CO2 that is susceptible to rapid acidification relative to other oceanic regions. However, the findings from this project suggest that the lagoon ecosystems may not follow this general pattern. More lagoon-specific research is necessary to gain a better understanding of this possible role reversal. Since about 50% of the Alaskan Arctic is made up of coastal lagoons, it is possible that new findings could change the estimates researchers are making about the magnitude of the Arctic’s CO2 sink.
The study underscores the need to expand high frequency measurements of ocean acidification variables in Arctic lagoons to provide insights into local drivers, species adaptations and the role of these water bodies in removing or adding CO2 to the atmosphere.
Recent Studies
In addition to these featured publications, studies are underway in the Arctic to see if temperature and ocean acidification can be a predictor of abundance of nearshore fish. Almost all of the global species response research on ocean acidification has been lab-based, so this research is novel in that it’s looking at species in the wild. There is also interest from the science community in better understanding the “benthic metabolism” or how the organisms that reside under the Arctic sea ice feed and produce CO2 and ultimately modify the pH of the water.
Diel Frequency of Nearshore pH Extremes: Miller and Kelley 2021
- 2017 – 2018 pH data recorded in Jakolof Bay and Bear Cove
Results
- Smaller inlet locations show a strong biological signal driving pH dynamics in spring and summer seasons
- Biological metabolism as well as tidal exchange were determined to be the main drivers of pH modification occurring daily
- More extreme conditions found in the more oceanic influenced Jakolof Bay
- Within these two locations, rapid hourly changes in pH were recorded demonstrating that these locations experience extreme physical and chemical environmental conditions on an hourly and daily scale.
Seasonal Phases of an Arctic Lagoon: Miller et al 2021
Seasonal Phases of an Arctic Lagoon: Miller et al 2021
- An autonomous pH sensor was deployed in Kaktovik Lagoon from 2018 – 2019 to capture two summer phases of open water (non-ice covered) and one closed phase (ice covered).
Results
- Patterns of pH variability from year-to-year during the open phase are not relatable.
- 2018, overall pH appeared to be higher and less sensitive to changes in salinity, whereas in 2019 salinity changes correlated with changes in pH.
- 2019 recorded an overall lower pH, CO2 emissions from the lagoon were positive whereas CO2 emissions in 2018 were minimally positive.
- This demonstrates that biological processes in the lagoon sediment and overlying water can be quite variable from year-to-year and dramatically affect whether or not the lagoon absorbs or emits CO2 to the atmosphere.
Abiotic Drivers of Arctic Nearshore Fish Abundance: Khalsa et al 2021
Abiotic Drivers of Arctic Nearshore Fish Abundance: Khalsa et al 2021
- High-frequency in situ measurements of pH, temperature, salinity, and dissolved oxygen were paired with daily fish catches in nearshore Beaufort Sea
- Generalized additive models (GAMs) were used to describe responses to nearshore environment for 18 fish species
Results
- Relationships between abundance and the physicochemical environment were variable between species and reflected life history.
- Each abiotic covariate was significant in at least one GAM, exhibiting both nonlinear and linear associations with abundance.
- Temperature was the most important predictor of abundance and was significant in GAMs for 11 species.
- pH was a significant predictor of abundance for 6 species: Arctic cod, broad whitefish, Dolly Varden, ninespine stickleback, saffron cod, and whitespotted greenling.
- Broad whitefish and whitespotted greenling abundance was positively associated with pH while Arctic cod and saffron cod abundance was negatively associated with pH.
Marine CO2 system variability determined from an Alaskan Ferry: Evans et al 2021
Marine CO2 system variability determined from an Alaskan Ferry: Evans et al 2021
- From 2017, the Alaska Marine Highway System M/V Columbia has served as a platform for surface underway data collection while conducting twice weekly ~1600-km transits between Bellingham, Washington and Skagway, Alaska.
- This 2-year dataset allowed for the assessment of marine CO2 system patterns along the Inside Passage. Surface water pH and aragonite saturation state (Ωarag) were determined using the pCO2 data with alkalinity from a regional salinity-based relationship, which was evaluated with discrete seawater samples and underway pH measurements.
Results
- Low pH and corrosive (Ωarag < 1) Ωarag conditions were seen during winter and in persistent tidal mixing zones;
- Corrosive Ωarag values were also seen in areas that receive significant glacial melt in summer;
- ‘Time-of-detection’ (how long it takes to calculate the rate of ocean acidification) was computed and revealed that tidal mixing zones may be sentinel observing sites with relatively short time spans of observation needed to capture the trend in seawater pCO2 equivalent to the contemporary 25 atmospheric CO2 increase.
- Anthropogenic CO2 was estimated and showed notable time and space variability. We theoretically considered the change in hydrogen ion concentration ([H+]), pH, and Ωarag over the industrial era and to an atmospheric pCO2 level consistent with a 1.5°C warmer climate and revealed greater changes in [H+] and pH in winter as opposed to larger Ωarag change in summer.
- In addition, the contemporary acidification signal everywhere along the Inside Passage exceeded the global average, with Johnstone Strait and the Salish Sea standing out as potential bellwethers for 30 biological OA impacts.
- In theory, roughly half the acidification signal experienced thus far over the industrial era may be expected over the coming 15 years with an atmospheric CO2 trajectory that continues to be shaped by fossil-fuel development.
Implications
Many aspects of ocean acidification research in Alaska are groundbreaking. This is uncharted territory during this unprecedented time in human history. Most nearshore data collection efforts throughout Alaska are currently working toward establishing a baseline for conditions so that we can observe and analyze changes over time. The trends and patterns that are beginning to emerge indicate that conditions are much more variable than we might have anticipated, and some species exhibit adaptations and resiliency that give us tentative hope for the future.
As regional conditions come into view, communities will be able to use carbon chemistry data to make better-informed decisions about natural resource management. For example, data tracking the variability of corrosivity in Kachemak Bay and Resurrection Bay can be used to associate the socioeconomic capacity of these waters for shellfish harvesting and aquaculture practices. New mariculture ventures may scout locations in areas of refugia. Fisheries managers may assess the benefits of pre-emptive human responses as fish abundance changes in higher latitudes. Most importantly, these various efforts to track ocean acidification along Alaska’s shoreline serve to create a robust profile of regional conditions that will allow stakeholders and policymakers to advocate for adaptive ecosystem-based management that includes response to major shifts in ocean conditions.
Ocean acidification is a continually growing problem driven by human emissions of CO2. The longer we continue along with business as usual, the more damage we will do to the marine environment and species we rely on and love.
Researchers
Partner Labs
Stories
Jun 2021
alaskafish.news
Apr 2018
Alaska Ocean Observing System